Bioprinting systems represent a groundbreaking advancement in tissue engineering and regenerative medicine, offering the potential to revolutionize the way we create biological tissues and organs for medical applications.
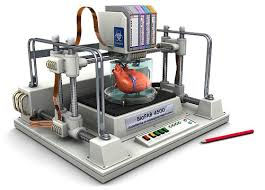
By employing 3D printing technologies, bioprinting allows for the precise layer-by-layer deposition of bioinks—materials that can include living cells, biomaterials, and growth factors—onto a substrate to create complex, three-dimensional structures that mimic the natural architecture of human tissues. This innovative approach addresses significant challenges in the field of organ transplantation and tissue repair, particularly the shortage of donor organs, the need for biocompatible materials, and the demand for customized solutions tailored to individual patients. With bioprinting systems, researchers and clinicians can create patient-specific tissues that are not only biologically functional but also capable of integrating seamlessly with the recipient's body, thereby reducing the risk of rejection and improving healing outcomes.
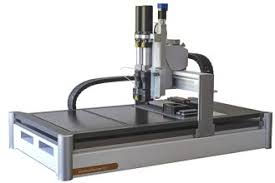
The bioprinting process begins with the selection of appropriate bioinks, which are crucial for the success of the printed tissues. Bioinks must possess specific properties, including printability, biocompatibility, and the ability to support cell viability and growth. They can be derived from various sources, including natural polymers like alginate, collagen, and gelatin, as well as synthetic materials that offer enhanced mechanical properties and tunable degradation rates. The choice of bioink directly influences the structure, functionality, and longevity of the printed tissues. Researchers are continually exploring new bioink formulations to optimize their mechanical and biological properties, leading to improved tissue quality and functionality.

The bioprinting process itself can utilize various techniques, each with unique advantages and applications. One common method is inkjet bioprinting, which employs thermal or piezoelectric forces to eject droplets of bioink onto a substrate. This technique is well-suited for creating high-resolution structures but may be limited by the viscosity of the bioink and the size of the cell aggregates. Another prevalent technique is extrusion-based bioprinting, where bioink is extruded through a nozzle to create continuous filaments. This method allows for the deposition of thicker bioinks and is effective for building larger structures. Laser-assisted bioprinting is yet another approach, utilizing lasers to create micro-droplets of bioink, enabling precise placement of cells and biomaterials in intricate patterns. Each method presents unique advantages and challenges, influencing the final tissue architecture and functionality.
One of the most significant applications of bioprinting systems is in the field of organ transplantation. The shortage of available donor organs for transplantation has led researchers to investigate the potential of bioprinting to create functional organs that can replace damaged or failing ones. By utilizing a patient’s own cells, bioprinting has the potential to generate organs that are immunologically compatible, thus reducing the risk of rejection. Research has shown promise in bioprinting various tissues, including skin, cartilage, bone, and vascular structures. For instance, bioprinted skin grafts have been developed for patients with severe burns, allowing for rapid wound closure and restoration of skin function. In the case of vascular tissues, bioprinting enables the creation of complex blood vessel networks that are essential for tissue viability and integration.
Comments